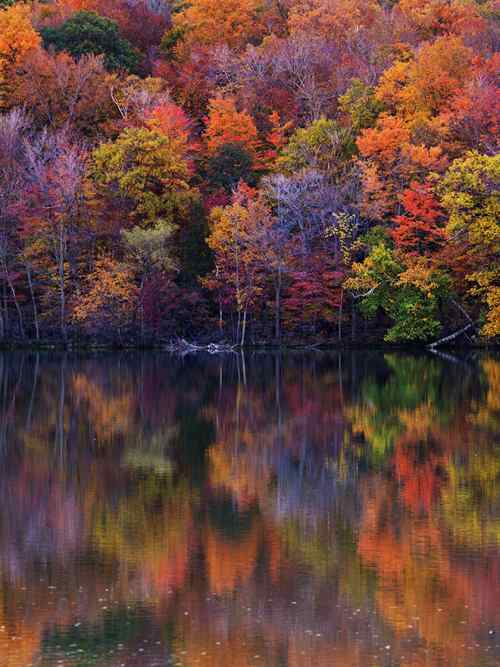
Quantum Advantage – Are We There Yet? (Part 3)
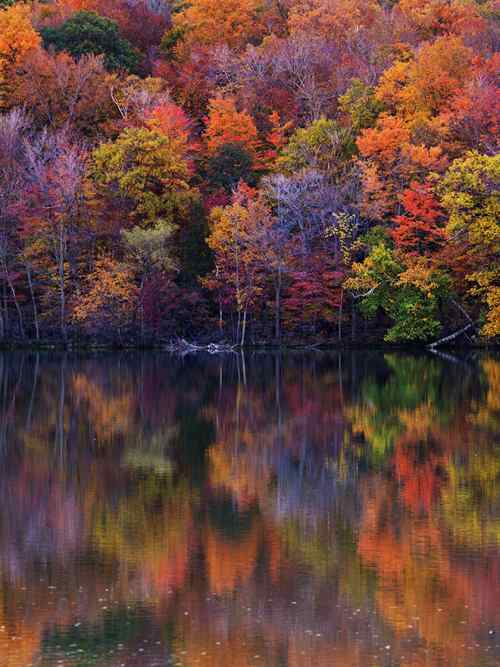
This is the third and final part of our three-part series exploring the quantum advantage that purports to accelerate drug discoveries, render current cryptographic techniques obsolete, turbocharge AI and machine learning, and basically solve the world’s problems. This part will explore the current state of the development of quantum computing technologies.
First past the post?
In October 2019, Google announced[9] that they had reached quantum supremacy with an array of 54 qubits, of which 53 were used, using what they call their « Sycamore » quantum chip. Google claimed that it performed a series of operations in 200 seconds which would typically take a classic computer 10,000 years to compute. If true, this would certainly be hard evidence of the power of quantum computers.
IBM countered,[10] stating that using existing supercomputers, breaking down the problem thrown at Sycamore and carefully optimizing the computer’s resources (i.e., memory) would take merely two and a half days to arrive at the result promoted by Google. In essence, IBM argued that although the Google experiment was faster, it had not in fact demonstrated quantum advantage. Still, even though this result by Google might not be an unequivocal demonstration of quantum advantage, it does underline that even a modest quantum computer can outperform a classical computer by a comfortable margin.
In December 2020, a group of Chinese researchers at the University of Science and Technology of China (USTC) announced[11] having also reached quantum advantage. In their results, the group claimed to have performed gaussian boson sampling[12] of 76 photons with their quantum computer named Jiuzhang[13]. The researchers state that obtaining the same number of samples that Jiuzhang obtained in 200 seconds would take a classical computer 2.5 billion years. Given that the Earth is more or less 4.5 billion years, one would probably agree that this is an unequivocal determination of quantum advantage – taking half the age of the earth to calculate something is a pretty long time.
USTC also very recently announced yet another milestone, claiming quantum advantage again, orders of magnitude higher than their previous result, and higher than the results published by Google in 2019[14].
The last two demonstrations by Chinese scientists have not been disputed as of yet.
But herein lies the crux of claiming quantum advantage: it is very much like beauty – it lies in the eye of the beholder. While Google can lay claim to having been the first to demonstrate quantum advantage, how you frame the problem to be solved and what hardware you use to solve it makes achieving quantum advantage a moving target. It is even possible that someone may hold the quantum advantage crown for a time, only to have the crown taken away by advances in traditional computing, requiring yet another demonstration of quantum advantage by a more powerful quantum processor. Or we may also have, say, one of the world’s top quantum scientists change their opinion that Google or USTC achieved quantum advantage, bringing the whole field back to square one[15].
Caveats
While we may draw conclusions about the power of quantum computers as they are today, the problems they are solving are experiments – the results are, for lack of a better word, useless. They are proof of concepts, not actually calculating a function with a usable result.
The quantum world is particularly sensitive to noise (interference from the outside world), and quantum systems are notoriously difficult to keep in a stable state for long periods of time.
A Canadian company, D-Wave is the first to have built and sell a quantum computer, although this computer works on the principle of annealing, which although very good, is not a “pure” quantum computer[16]. D-Wave’s computer requires that the qubits themselves, and a lot of the electronics that connect to the qubits, need to remain in a very cold environment. The coldest temperature recorded on Earth was in 1983, at the Vostok Station in Antarctica, where it was a freeze-your-eyelids-together-in-no-time -89.2oC! However, even that is not cold enough for D-Wave’s core, which needs to be chilled to -273.13oC, or a hair above absolute zero. At this temperature, the core is in a stable environment long enough to be able to perform calculations. Cooling, and maintaining the core at this temperature adds significant cost to the compute cost of quantum computers, making them for the time being out of reach for most companies.
Quantum computers are also prone to errors and schemes have been proposed to error-correct results on the go. Such schemes require using (for now) a significant number of qubits for error correction, which although increasing the reliability of the results, reduces calculation speeds.
Conclusion
While there have been significant advances in the realm of quantum science, and quantum computing, with at least a theoretical demonstration of quantum advantage, it does not appear that we are on the verge of a commercially viable quantum computer able to solve problems that have a useful result. Experts’ predictions on when that might happen range from sometime in the next 2-5 years, to never. There are still significant engineering and scientific hurdles to overcome, although progress is being made at what sometimes feels like breakneck speed.
In other words, the cryptography schemes that are used today to secure almost all communications are not yet susceptible to being broken by a quantum computer, but we should probably prepare, just in case.